Cycling-Induced Degradation of Batteries
Rechargeable batteries solve electrification and communication problems. As examples, hybrid battery-diesel generator power supplies efficiently power cell towers in remote locations, detached from the power grid. Large battery banks are used to load level user power requirements, reducing stress on power generation infrastructure. Batteries firm the output capacity of intermittent wind-turbine and photo-voltaic power sources.
High-temperature, sodium–metal chloride battery cells are used in each of these applications. These cells deliver 2.58V open-circuit potential. The failure mode is benign, and the failed cells perform as low-resistance links, so that cells wired in series continue to perform in the presence of one or more failed cells. The cell features a molten sodium negative electrode and a mixed transition-metal-chloride positive electrode, separated by a ceramic, sodium-ion-conducting electrolyte.
Sodium – (nickel, iron) chloride rechargeable battery cells were built with thin, annular, packed-bed cathodes; sandwiched between cylindrical molybdenum current collectors and cylindrical, sodium-ion conducting alumina tubes. The sodium anode forms during charging in the volume between the outside of the alumina tube and the inside of the steel case. Figure 1 is a computed-tomography image of a cell. The cell was designed specifically for our study; it differs from commercial cells.
Cells were repeatedly deeply discharged at low, constant power and charged at constant power, then constant voltage. Cycling resulted in accelerated degradation. Figure 2 shows the progression of the voltage curves.
Cell electrochemical performance was modeled with one-dimensional, axisymmetric partial-differential equations, using porous electrode theory; and the model was solved with time-dependent, finite-element methods using the COMSOL Multiphysics® software.
Cell performance was simulated, as built, and at various times after degradation had occurred. Materials parameters were adjusted for each simulation to fit the evolving voltage-current-time response of the degraded cells, charging and discharging.
The key change associated with cycling degradation, as predicted by model, was the gradual loss of electrochemical participation of iron and a fraction of the sodium chloride. The rates of participation loss for these positive electrode components are shown in Figure 3.
Selected cells were quenched in the discharged state, and disassembled. The top third of the cathode beds had lost their granular structure. X-ray diffraction analysis revealed that the top of the bed was depleted of nickel and metallic iron, but rich in iron(II)chloride and Na6FeCl8 (“shifted salt”). These salts, formed by electrochemical oxidation of iron, should only occur in charged cells. Their presence in discharged cells indicates that they are no longer electrochemically active. Thus, modeling predictions were supported by microstructural evidence. Although accelerated degradation testing was performed in a purpose-built cell design, the learnings are applicable to real-world battery applications.
We acknowledge the financial support from the New York State Energy Research and Development Authority (NYSERDA) via the New York Battery and Energy Storage Technology Consortium (NY-BEST). NYSERDA has not reviewed the information contained herein, and the opinions expressed in this report do not necessarily reflect those of NYSERDA or the State of New York.
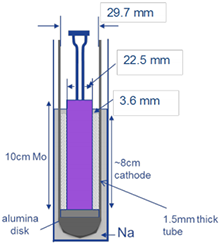
ダウンロード
- vallance_presentation.pdf - 1.3MB
- vallance_abstract.pdf - 0.1MB